Sunday Science: A Strange Fascination
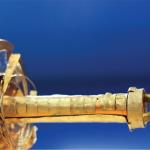
Something strange is afoot in Silke Bühler-Paschen’s lab at the Vienna University of Technology. The walls of the room are plastered in copper foil to keep out electromagnetic waves. A blue refrigerator dangles through a hole in the ceiling, suspended from robotic shock absorbers that precisely counteract the slightest vibrations, including from subway cars passing deep underground. Condensation drips down the fridge into a Minion-themed kiddie pool. Inside, a hair-thin sample of an exotic material is cooled to thousandths of a degree above absolute zero. What happens within this material, and the way it conducts electricity, is one of the biggest mysteries in condensed matter physics.
The electrons begin their journey through Paschen’s lab from an ordinary wall outlet. According to the standard theory of electricity, they migrate individually or in small clusters through the wires leading to the refrigerator. But once the electrons reach the sample—a compound of ytterbium, rhodium, and silicon—this simple picture breaks down. The sample belongs to a class of materials that physicists call “strange metals.” For 4 decades, they’ve puzzled over the fact that in these compounds, the standard theory of electricity just doesn’t work.
Recent experiments in Paschen’s lab and others suggest that in strange metals, electrons lose their individuality. “They magically disappear,” she says. Instead, electric charge appears somehow to pass through the metal as a diffuse amorphous blob—like water without individual H2O molecules. Researchers are still debating the microscopic details of this bizarre picture. But it’s already clear that the stakes are higher than just understanding a dozen or so oddball materials. “It’s really a mysterious state with big consequences,” Paschen says.
The hallmark of strange metals is electrical resistivity that climbs higher than that of ordinary metals when they are warmed from low temperatures. They also lose their resistivity altogether, becoming superconductors, at lower temperatures—though above those of conventional superconductors. Some researchers believe this high-temperature superconductivity is simply the flip side of strange metallicity—that they’re two manifestations of the same underlying phenomenon. If so, then the path to room-temperature superconductors, a long-sought goal that could revolutionize technologies from power grids to transportation, may lead through an understanding of strange metals. “You have to get strange metals right before you can get superconductivity right,” says Philip Phillips, a physicist at the University of Illinois Urbana-Champaign (UIUC). “That’s the heart of it all.”
But the implications go beyond just building better superconductors. A theory that explains strange metals may force a fundamental rethinking of how electricity works in all materials. It might subsume the standard theory the way general relativity, with its curved spacetime, subsumed Isaac Newton’s theory of gravity—and prove just as unsettling. Strange metals are forcing physicists to ask whether the very idea of an electron, or any particle for that matter, is an oversimplification of what’s really going on. “The violation of the standard theory of solids in these strange metals is so dramatic—it’s in your face,” says Qimiao Si, a physicist at Rice University who collaborates with Paschen. “There’s no question there’s new physics.”
That prospect has energized researchers. “The strange metal problem is the hardest problem we have in condensed matter physics,” says Peter Armitage of Johns Hopkins University. “Since when do physicists run away from hard problems?”
RISE OF THE QUASIPARTICLE
The theory of electricity in solid materials has been overhauled before. For a few decades after electrons were discovered at the end of the 19th century, physicists treated them as independent particles that passed through the lattice of atoms in a conductor like pinballs, scattering off the atoms. Researchers knew reality was more complicated—that electrons also repel one another because of their negative charge—but calculating the effects of zillions of such interactions was unfeasible.
In 1956, Russian physicist Lev Landau found a shortcut: He could account for at least some of these electron interactions by treating a clump of electrons as a single heavier particle called a “quasiparticle.” It wasn’t a physical particle—it was a state of excitation shared by many particles, like a wave of sports fans standing up in a stadium. But mathematically, quasiparticles act like conventional particles coursing through a metal, scattering off atoms and one another. That scattering generates electrical resistivity, and quasiparticles made it possible to calculate resistivity more accurately. Landau’s model, called Fermi liquid theory, remains the canonical understanding of how electricity flows through solid materials.
The model captures the properties of metals on the periodic table remarkably well. That is, apart from superconductivity. In 1911, when physicists at Leiden University used liquid helium to cool solid mercury below 4°C above absolute zero, its resistivity suddenly dropped to zero. Landau’s model alone couldn’t explain this behavior, but just 1 year after he proposed his theory, physicists came up with a workaround.
Called BCS, the theory holds that at such low temperatures, electrons trigger vibrations in the superconductor’s atomic lattice that effectively glue pairs of electrons together, in spite of their electrostatic repulsion. These Cooper pairs (named for one of the authors of BCS theory) then settle into their lowest energy state. In that state they can’t scatter off the lattice, because that would require them to lose even more energy. So they flow through the material resistance-free.
This picture worked fine for decades. But it was challenged again in 1987 when physicists at IBM in Switzerland found that certain copper-based compounds, or cuprates, could superconduct about 30°C warmer than liquid helium—too hot for the BCS mechanism to operate. This time, there was no quick fix. To this day, scientists haven’t identified the extrastrong “glue” that could be binding electrons together at such high temperatures. As they have continued to discover materials that superconduct at higher and higher temperatures, the mystery has grown more acute.
Silke Bühler-Paschen holds a strange metal made of cerium, palladium, and silicon. She found evidence that its electrons are quantum-mechanically entangled. Photo: Matthias Heisler/vienna University of Technology
Yet a clue lurked in the original experiments at IBM. When the scientists heated their cuprate sample above the critical temperature, they noticed another peculiar feature: The resistivity rose in a straight line instead of along an exponential curve, as it does in all other known metals. Fermi liquid theory could not explain this odd behavior. Furthermore, in some materials, the resistivity continued to rise steadily instead of flattening out as the temperature climbed further (making them particularly bad conductors). To explain such high resistance in Landau’s framework would require electron quasiparticles to be scattering at distances shorter than there are things to scatter off, in the empty spaces of the atomic lattice.
Gradually, physicists began to realize the unusual resistivity of these “strange metals,” as these materials came to be called, was just as important a puzzle as their high-temperature superconductivity. “It has the hallmark of a really good physics problem: It’s very simple, and it seems to require a big conceptual change,” says Peter Abbamonte, a physicist at UIUC.
In 2004, Dutch physicist Jan Zaanen noted something else about resistivity in strange metals. The slope of a material’s resistivity is a measure of how fast it dissipates an electrical current as heat. In normal metals, the electron scattering rates depend on microscopic details of the material. But in strange metals the dissipation—hence the resistivity—always seems to rise at the fastest possible rate. Moreover, that rate is proportional to Planck’s constant, a key value in quantum mechanics that determines how precisely certain properties of particles can possibly be measured. “Planckian dissipation,” as Zaanen dubbed it, implied that the behavior of electrons in strange metals must reflect blurry quantum effects that aren’t accounted for by Landau’s quasiparticles.
By 2019, Planckian dissipation had turned out to be a general property of cuprates. That year Zaanen drafted a 40-page manifesto challenging his colleagues to consider linear resistivity as the “expression of a new, truly fundamental physics” that required them to kill the quasiparticle model.
Theorists are now trying to do just that. In a review in Science in 2022, a team led by Phillips concluded that in strange metals, at least, “electrons are no longer the primary charge carriers. When the particle picture breaks down, no local entity carries the current.” The question is what does.
THE SILENT PLACE
For a long time many physicists resisted Zaanen’s call to arms. Phillips was not one of them. “Quasiparticles were always a crutch, and we need to throw away the crutches,” Phillips says. Lately a flurry of new experimental techniques has begun to highlight all the strangeness that Landau’s simplified picture had overlooked.
On sabbatical at Rice in 2016, Paschen teamed up with Si and his colleague Doug Natelson to concoct a way to, in effect, listen to the current inside strange metals. Just as recording the pitter-patter of rain on your roof can tell you about the size and frequency of raindrops, measuring the fluctuations in current along a strange metal wire can tell you about the nature of whatever is carrying the current. Rather than picking up the inconsistent crackling of passing electrons or quasiparticles, the noise the researchers heard was dead consistent. Electricity seemed to be flowing through the wire as a homogeneous soup.
The results, reported in 2023, dealt the strongest blow yet to the notion of quasiparticles. Paschen says the experiment totally reframed her mental image of what’s going on inside a strange metal. In her presentations, she used to represent it as a chaotic tornado of messy electron interactions. Now, she thinks “it’s actually something very controlled. It’s the silent place.”
Other researchers have found ways to probe the properties of strange metals more directly. Armitage shines far-infrared light at samples and finds no evidence for quasiparticles. Stephen Hayden, a physicist at the University of Bristol, shoots a beam of neutrons at them and finds waves of magnetism that slow down as the sample cools, a hint that the material is undergoing a transition to a new state. Abbamonte uses an electron gun to explore variations in the density of electrons in the material, and finds instead a uniform spread of charge. “There’s no measurement you can do with the system that tells you how many electrons are in it,” Abbamonte says. “They really just behave in a very bizarre way.”
All three of these recent scattering experiments also suggest different properties of electrons in these strange metals are “scale invariant.” Measure, for instance, fluctuations in the charge density as a function of temperature, and it will follow the same general curve at a narrow temperature range as at a wide one. Observing the physical phenomena inside a strange metal is like zooming in on a snowflake: Things look the same at all scales.
Meanwhile, physicists are seeing hints of the same strange electron behavior in materials very different from the original cuprate compounds, from rotated sheets of graphene to starshaped lattices of nickel and indium. The challenge to the existing picture of electricity seems to extend far beyond a few outlier metals.
In 2023, Zaanen fell into a coma after treatment for his esophageal cancer— a consequence of a lifelong devotion to cigarettes, one that transcended mere addiction. (“Nicotine fueled his mathematics,” Abbamonte says.) When he woke up in the hospital, his family told him they were already planning his memorial service. He asked whether he could go. That summer, Zaanen threw his own funeral in Leiden, and physicists flew in from around the world to join the party. He died a few months later.
“All of us feel an urgency to get this problem solved, and it’s in no small way because he died,” says Phillips, who considered Zaanen among his closest friends. “We owe it to Jan to solve this problem.”
REWIRING ELECTRICITY
Landau’s theory is so ingrained that physicists aren’t sure how to talk about electricity without it. “The words we use very often presuppose that we have these electronic quasiparticles,” Armitage says. “It may be that a different lens is needed, but what that is we don’t know.”
Zaanen’s manifesto left some hints. He proposed that at the center of strange metal behavior is “entanglement,” the quantum phenomenon that links properties of particles, allowing them to act almost as a single object, even when they’re too far apart to communicate. “We are dealing with a completely new form of matter controlled by a system where literally everything is entangled with everything,” he wrote.
Maximally entangled electrons would form a diffuse “liquid” that would have the lowest possible viscosity, according to Zaanen. Imagine dropping a rock into a soup—the thinner the broth, the faster the ripples will dissipate. Because the quantum soup has minimal viscosity, it will dissipate energy at the fastest possible rate, explaining why the resistivity of a strange metal rises so fast as it’s warmed. He stopped short of explaining how this entangled electron soup might form. But three theorists are now putting forth distinct attempts.
The first comes from physicist Subir Sachdev of Harvard University. Three decades ago, he helped develop a mathematical model of how random interactions of electrons lead them to become entangled. In a Science paper in 2023, Sachdev used an updated model to argue that in strange metals, quasiparticles scatter off magnetic waves and imperfections in the atomic lattice and break apart, leaving a highly entangled soup of electrons.
Hayden says Sachdev’s theory “seems to have a good stab at explaining what we see” in his recent neutronscattering experiments, particularly the behavior of the magnetic waves. Paschen finds the theory’s mathematical and explanatory power “very appealing,” but she doesn’t buy the importance of randomness and lattice imperfections in driving entanglement. Many strange metal samples, including her own, are exquisitely pure and regular, she says.
Last year, Paschen found a way to actually probe the entanglement inside a material by following an approach proposed by Peter Zoller of the University of Innsbruck in 2016. With Si and Fakher Assaad at the University of Würzburg, she used the technique to estimate the minimum amount of entanglement present in a strange metal made of cerium, palladium, and silicon. Posted as a preprint in March, their result—at least nine entangled electrons—sounds tiny, but it’s an extremely conservative estimate, Paschen says, and also the strongest entanglement ever documented in any solid-state system.
The experimental confirmation of multiple-particle entanglement led Si to a second theory of strange metals, published in Nature Communications in March. Si invokes interactions between two sets of electrons in the metal: the conduction electrons that can move freely, and the inner electrons that are locked to metal atoms. In normal metals, the conduction electrons and the spins of inner electrons link to form quasiparticles. But in the strange-metal state, the inner electrons are so strongly entangled among themselves—as evidenced by Paschen’s experiments—that they’re restrained from talking with the outer electrons. This leads to a breakdown of the quasiparticles, leaving a maximally entangled blob of conduction electrons that passes through the lattice only with difficulty, Si says—hence the high resistivity. But if the material is cooled below its critical temperature, the agitated electron soup will reorganize into a superconducting state.
Silke Bühler-Paschen’s lab in Vienna has copper-clad walls to shield it from electromagnetic noise that could drown subtle signals from strange metals. Photo: Duy Ha Nguyen/vienna University of Technology.
Paschen plans to explore the transition Si has proposed by using her big blue fridge to run her noise experiments at lower and lower temperatures. In a sparkly white clean room, her team is busy spraying material samples onto thin films. Once they cut these samples into wires and load them in the chamber, Paschen will listen to the noise in hopes of hearing a change of entanglement in the material as it transitions from strange metal to superconductor.
Si says the ability to measure entanglement is a breakthrough that’s already yielding new insights. “I think there’s potentially a floodgate that’s being opened up,” he says. Phillips agrees. “It’s a really, really big deal.” In a preprint in March, he used a similar approach to calculate the level of entanglement observed in his colleague Abbamonte’s electron-scattering experiment. He concluded it was significantly larger than in normal metals. Furthermore, Phillips believes Abbamonte’s experiment is evidence that whatever carries charge in strange metals doesn’t have a well-defined mass or energy. This belief underlies his third explanation for strange metal behavior.
Over the past decade, Phillips has argued that current in strange metals is carried by something radically different from electrons, even entangled ones. He favors what Harvard physicist Howard Georgi called “unparticles”—a mind-bending, still hypothetical form of matter. Unlike all known particles, which have a well-defined mass at rest, an unparticle could take on any possible mass, depending on how it’s measured. Phillips says a soup of variable-mass unparticles is the only way he can make sense of all the bewildering experimental data on strange metals. But he’s not sure yet how that soup would give rise to strange metals’ hallmark—their linear resistivity.
Phillips acknowledges that his idea is further out than Sachdev’s or Si’s. “I seem to be fundamentally incapable of doing anything that is well accepted,” he says. As a Black physicist, he explains, he’s grown accustomed to the experience of not fitting in. “It’s easier to go against the grain when you are not part of the grain,” Phillips says.
Inside this machine, Silke Bühler-Paschen’s team sprays an ultrathin strange metal film onto a substrate to be used in her experiments. Photo: Z. Savitsky/science
Yet his distaste for particles does fit a trend that extends outside the strange metal community, says Meigan Aronson of the University of British Columbia, who studies how new kinds of matter emerge near quantum phase transitions. “We learned about electrons in school, so we’re all very attached to them,” Aronson says. But over the past few decades, breakthroughs in the field have repeatedly shown how the collective behavior of electrons can explain phenomena that individual electrons can’t. Like Phillips, Aronson suspects that “maybe electrons are not the fundamental particle in condensed matter physics.”
Zaanen would have approved. “Twentieth-century physics revolved around the particle idea,” he wrote in his manifesto. But when it comes to strange metals, “it cannot be emphasized enough how misleading the very idea of a particle is.”
THE GLUE IS SOUP
In April, at a workshop at the Max Planck Institute for the Physics of Complex Systems in Dresden, Germany, a handful of strange metal enthusiasts assembled to compare their new ideas. Although the theorists retain their healthy rivalry, “We’re all going in the same direction,” Si says. He, Sachdev, and Phillips all agree that current flows through strange metals as a diffuse quantum soup lacking any localized electron quasiparticles. They just each have different ideas about the exact ingredients of that soup.
” feel we’re pretty close to understanding strange metals,” Sachdev says. “The ideas are not so far from each other—we’re talking about the same elephant from different points of view.”
The theorists are also united in their conviction that the new insights from strange metals are the key to making better and higher temperature superconductors. For decades, investigators have focused on figuring out the “glue” that might bind electrons into Cooper pairs at higher temperatures. But if charge actually travels as some entangled quantum blob, that effort may be misguided. “You don’t need glue if the whole thing is a soup,” Si says.
The way to find revolutionary, ambient-condition superconductors, Si says, is to look at materials that are particularly bad conductors at higher temperatures. “It’s mind-boggling, but that’s the strange metal paradigm,” he says. Si thinks the high resistivity of strange metals indicates electrons in this state are “frustrated,” and when cooled enough they naturally reorder into a more comfortable superconducting state. His goal is to lift that transition to room temperature: “You make these particles as unhappy as possible so that they can reorganize into something that we actually care about for certain purposes, such as saving the world.”
For his part, Phillips thinks unparticles could be the path not just to room-temperature superconductors, but to rethinking electricity without the electron. At the Dresden workshop, he filled a blackboard with math describing how to replace quasiparticle descriptions with unparticle ones. “You have to go and show in your theory how the quasiparticle is killed,” he says. “If you kill the quasiparticle, you have a new starting point, and that’s what we’ve all been trying to find.”
An extracurricular passion far removed from physics fortifies Phillips for this creative destruction. At 3:30 p.m. on the dot, he excused himself from the workshop: It was time for opera rehearsal. In a windowless office kitchen with cherry trees blooming outside the windows, Phillips unleashed his powerful bass. Tea mugs trembled as he recited a song, in its original German, from Mozart’s The Abduction from the Seraglio. One line stood out: “I study day and night, I rack my brains, and I won’t rest until I see you killed.”
Quasiparticles are on notice.
Zack Savitsky is a journalist specializing in the physical sciences.
Science has been at the center of important scientific discovery since its founding in 1880—with seed money from Thomas Edison. Today, Science continues to publish the very best in research across the sciences, with articles that consistently rank among the most cited in the world. In the last half century alone, Science published:
- The entire human genome for the first time
- Never-before seen images of the Martian surface
- The first studies tying AIDS to human immunodeficiency virus
A trailblazer in online publishing as well, the Science family of publications has grown to include online journals Science Translational Medicine, Science Signaling, Science Immunology, Science Robotics and the open access journal Science Advances.
NIH killed grants on orders from Elon Musk’s DOGE
Max Kozlov
Nature
Court documents and internal correspondence show the cost-cutting force has broad control over the world’s largest public biomedical funder.
May 21, 2025